Advancing Regulatory Framework for Predicting the Proarrhythmic Risk
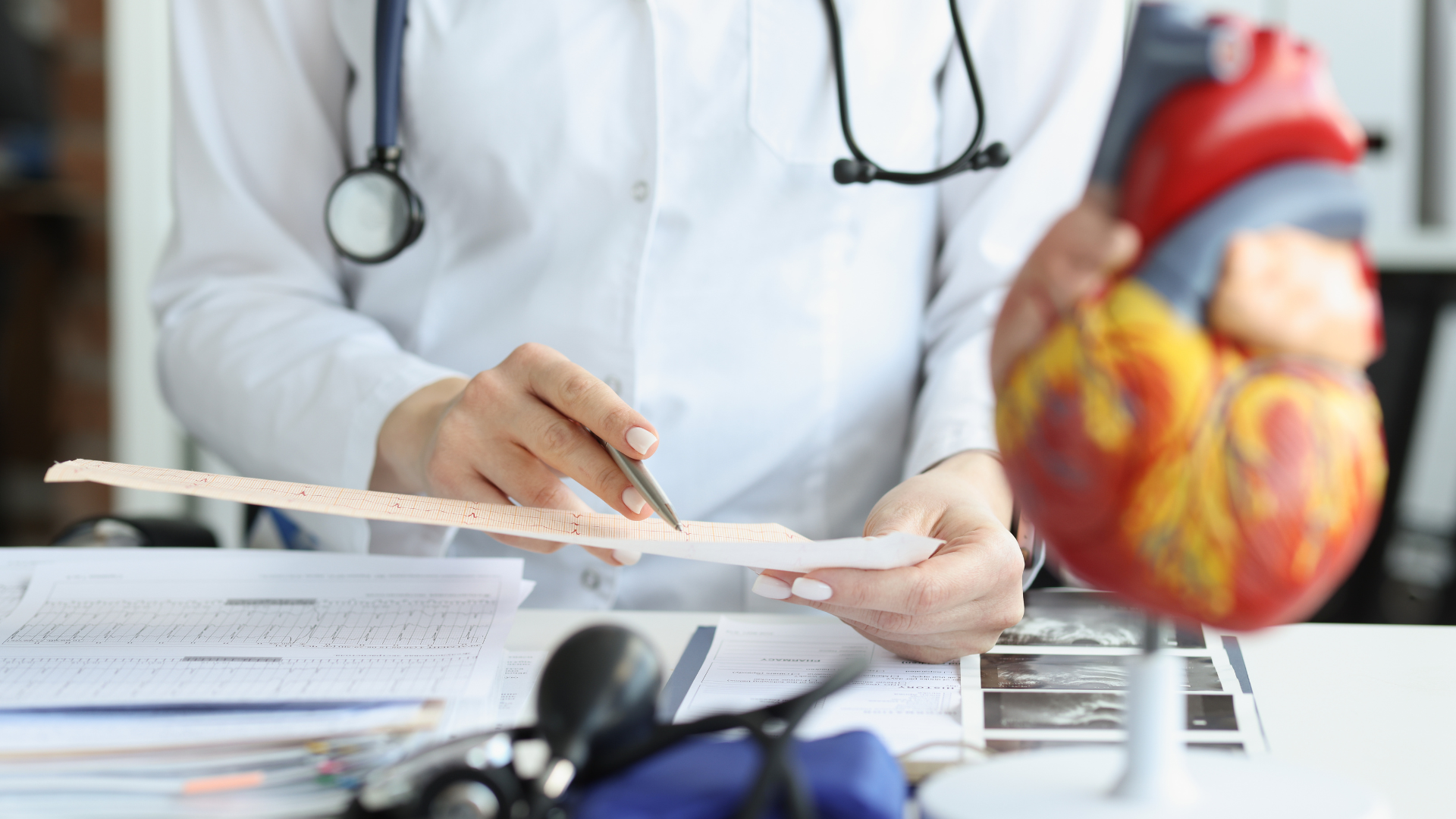
The issue of QT prolongation has garnered significant attention from regulatory bodies such as Health Canada, the Food and Drug Administration (FDA), and the European Medical Agency (EMA). Back to the 1950s, doctors observed an alarming phenomenon among some heart patients who were being treated with quinidine. These patients experienced fainting spells, convulsions, and, in some tragic instances, sudden cardiac death. Electrocardiograms (ECGs) of these individuals revealed an abnormal and rapid heart rhythm, characterized by a distinctive spiked pattern known as “TdP” (Torsades de Pointes).
The QT interval on an electrocardiogram (ECG) represents the time between the start of the Q wave and the end of the T wave. It reflects the time taken for ventricular depolarization and repolarization. QT prolongation is a condition where the repolarization phase of the heart’s electrical cycle takes longer than usual. This delay in cardiac repolarization can increase the risk of dangerous arrhythmias like Torsades de Pointes (twisting of the points, TdP), a condition that can escalate into ventricular fibrillation and ultimately lead to sudden death. The recognition of these risks has led to the implementation of Thorough QT or TQT studies in drug development to assess the potential for QT prolongation.
Fast forward to the 1990s and early 2000s, and the medical community faced a similar issue with certain medications. Several drugs were withdrawn from the market during this period due to concerns about their potential to trigger a fatal arrhythmia, including Torsades de Pointes. Notable examples of these drugs include Cisapride and Terfenadine.
Cisapride, initially marketed as Propulsid in the United States, received approval primarily for treating nighttime heartburn related to gastroesophageal reflux disease. However, it found widespread off-label use for various conditions, such as diabetic gastric atony and peptic ulcers. By December 31, 1999, there had been 341 reported cases of heart abnormalities and 80 deaths associated with cisapride use, especially in patients simultaneously taking medications that inhibited its metabolism. This unfortunate situation highlights the challenge of linking QT prolongation to unexpected sudden deaths and underscores the need for proactive regulatory measures including TQT studies, to better understand these risks.
Similarly, Terfenadine, an antihistamine available both over-the-counter (OTC) and by prescription, faced a comparable outcome. It gained notoriety due to its potential to induce severe cardiac arrhythmias, including TdP, particularly when taken in high doses or in combination with certain medications. Consequently, many countries withdrew terfenadine from the market during the 1990s, and it has since been largely replaced by safer alternatives among antihistamines.
These cases underscore the serious risks associated with these medications, as they had the potential to prolong the QTc interval, leading to potentially life-threatening cardiac arrhythmias. The situations with both Cisapride and Terfenadine, as well as other drugs known to carry QTc risks (such as certain antiarrhythmics, antibiotics like macrolides and fluoroquinolones, antipsychotics, and others), highlighted the imperative need for regulatory action including the implementation of TQT studies, to prevent such adverse outcomes.
Evolving regulatory paradigm for proarrhythmic risk assessment
1. From 2005 to 2010:
Nonclinical and clinical regulatory guidelines, most notably ICH S7B and ICH E14 that currently govern the cardiac safety landscape, were first released in 2005, reinforcing the importance of identifying the liability for QT prolongation.
The S7B guideline provides a nonclinical testing strategy to evaluate the potential for human pharmaceuticals to affect cardiac electrophysiology, with specific attention focused on ventricular repolarization. The 2 highlighted biomarkers of particular interest are the repolarizing ionic current IKr and the QTc interval (the QT interval “corrected” for the effects of heart rate).
The ICH E14 Guidance addresses clinical evaluation of QTc prolongation and the proarrhythmic potential of nonantiarrhythmic drugs. The document describes a dedicated clinical trial, the Thorough QT (TQT) study, may be warranted to assess the degree to which a drug compound, having systemic bioavailability to be indicated for uses other than the control of arrhythmias, affects the QT duration when nonclinical studies suggest a potential for QT prolongation or early phase clinical studies raise concerns.
The “classic” TQT study is a randomized, double-blind crossover research featuring four arms: placebo, active control, and two study drug doses. An active control arm (often moxifloxacin) gauges sensitivity to detect QT prolongation and must demonstrate the appropriate QT changes. Both therapeutic and supratherapeutic drug exposures should be tested, mimicking real patient scenarios. The TQT aims to detect even minor QT prolongations, typically around 5 milliseconds (ms).
Conducting the TQT study requires a substantial amount of compound information, including what constitutes a therapeutic and supratherapeutic dose; thus, it’s not initiated too early in the development process. When such prolongation is identified, extensive ECG collection and analysis in later-stage studies follow, incurring significant costs. Most drug developers discontinue compounds with positive TQT results unless benefits far outweigh QT prolongation risks and the risks are clearly presented in the product label.
Since 2005, around 450 TQT studies have been carried out, incurring costs exceeding $1 billion for drug developers. Despite the mandatory nature of these tests, no new drugs have been withdrawn from the market due to arrhythmia risks. Nevertheless, it remains uncertain whether the TQT approach effectively identifies only those drugs that genuinely pose a risk.
From a certain perspective, both the S7B and E14 guidelines have been successful because they have prevented the withdrawal of marketed drugs on TdP grounds since their adoption. However, it’s important to acknowledge some significant limitations. Notably, while increases in the heart rate-corrected QT interval (QTc) are highly sensitive, they lack specificity when it comes to predicting the risk of ventricular proarrhythmia.
While the initial focus on evaluating new drugs for their potential to block the hERG potassium channel and extend the QTc interval has been successful in preventing the introduction of TdP-inducing drugs to the market, it has also resulted in the premature abandonment of certain drug candidates during their development, sometimes unjustifiably. This is because not all drugs that block the hERG potassium channel and lengthen QTc actually trigger TdP, often due to their interactions with other channels. A TQT study in this context can help differentiate between drugs that are likely to induce TdP and those that are not, despite their effect on the QTc interval.
It’s important to note that drugs which prolong the QTc interval, often by blocking the hERG current at therapeutic plasma concentrations, do not necessarily lead to proarrhythmia. Examples of such drugs include ranolazine, phenobarbital, and tolterodine. Verapamil, while a potent IKr blocker, does not typically cause QT prolongation, except possibly at very high intravenous doses, primarily due to its simultaneous inhibition of the calcium current. Amiodarone serves as an example of a drug that significantly prolongs the QTc interval, sometimes exceeding 550 milliseconds, yet very rarely induces TdP.
Additionally, the degree of QTc prolongation, except in cases of drugs primarily blocking the IKr channel, varies significantly depending on the specific drug. Many factors unrelated to proarrhythmia, such as dietary habits, concomitant medication use, autonomic fluctuations, glucose and insulin levels, and circadian rhythms, can also contribute to QTc prolongation.
When the prolongation of the QTc interval reaches a level of regulatory concern, it typically requires extensive ECG assessments during phase 3 trials, including a TQT study as per the regulatory framework established in 2005. This can present a substantial hurdle in the progression of drug development and often results in cautious labeling of the product.
The prevailing perception that even a minor impact on the IKr channel or mild QTc prolongation can trigger adverse regulatory and commercial consequences during the drug development process can create substantial obstacles, particularly for smaller companies striving to innovate and develop new compounds to address unmet medical needs.
As a result, the regulatory paradigm put forth in 2005 may inaccurately attribute TdP liability to certain drugs. Hence, there may be a need for this paradigm to evolve to better predict the risk drugs pose to the heart.
2. From 2010 to 2020:
Both Crossover and parallel designs work for assessing QT interval prolongation. Crossover studies need fewer subjects, acting as their own controls, reducing variability. They’re good for heart rate correction based on individual data. Parallel studies are preferred for drugs with long half-lives or carryover effects due to irreversible binding or active metabolites, especially when comparing multiple doses or treatment groups.
In 2010, the FDA advocated a change in prolonged QT interval assessment approach for parallel design, suggesting combined placebo/moxifloxacin arms in parallel studies rather than separate arms, which allowed for a more precise assessment of moxifloxacin and study drug effects on QT intervals. The FDA also reconsidered heart rate correction methods, moving away from Bazette’s correction (QTcB), which had been used since the 1920s but tends to overestimate QT duration at heart rates above 60, into Fridericia’s correction (QTcF) utilizing the cube root of the heart rate interval, which offers similar accuracy but at a lower cost than individual heart rate correction (QTcI) the FDA initially recommended.
ICH E14 initially questioned the validation of ambulatory ECG monitoring as the primary method for assessing QT/QTc intervals. However, data showed that both 12-lead Holter monitors and standard 12-lead ECG machines yield similar results. Holter monitors are now the standard for ECG data collection in Thorough QT (TQT) trials, as they simplify study conduct and reduce data loss due to poor acquisition. ECG data review methods have also evolved, shifting from manual reading of paper recordings to digital tracing reviews on computer monitors, with increasing automation to improve measurement consistency and reduce variability.
As mentioned earlier, the “classic” TQT study is a randomised, double-blind research to minimise bias and a crossover study is preferred. The four treatments recommended are placebo, active control and two doses of study drug. The positive control arm (usually moxifloxacin) is used to show that the study is sensitive enough to pick up QT prolongation around the threshold of regulatory concern (Philip, et al., 2014). The study was designed to exclude a QT effect above the threshold of concern, i.e., an effect exceeding 10 ms must be excluded at all postdose timepoints. In case such a “threshold” effect cannot be excluded, the implications in terms of ECG monitoring in late-stage trials are substantial and if the drug is approved, the labeling will include appropriate recommendations.
Recognizing the costs and additional time required for the TQT study, the regulators and its scientific collaborators sought to explore whether it was possible to measure QT prolongation in the Phase 1 study, which involves administering escalating doses of a drug to a small number of participants, instead of conducting a separate TQT study.
Members of the ICH E14 Implementation Working Group (IWG) proposed the need for prospective data to ascertain the reliability of Phase 1 concentration-response analyses in assessing a drug’s impact on QTc intervals, potentially eliminating the necessity for a separate study with a positive control. To address this, the International Consortium for Innovation and Quality in Pharmaceutical Development – Cardiac Safety Research Consortium (IQ-CSRC) conducted a study specifically designed to prospectively evaluate the feasibility of utilizing concentration-response relationships in a Phase 1-like setting.
The IQ-CSRC study, a third-party blinded, randomized, placebo-controlled trial involving 20 healthy volunteers, mirrored the design of a Single Ascending Dose (SAD) Phase 1 study. Employing a linear mixed-effects model, the study analyzed change-from-baseline QTc (QTcF) as the dependent variable, with drug plasma concentration as a continuous covariate and nominal time post–first dose as a factor. The analysis criteria were pre-specified for positive and negative QT assessments, designating a “QT-negative” drug as having an upper bound of the 2-sided 90% confidence interval of predicted placebo-corrected QTcF below 10 ms at the observed geometric mean Cmax level of the supratherapeutic dose. All five drugs known to prolong QT met the criteria for a positive QT assessment, while the non-QT-prolonging drug satisfied criteria for a negative QT assessment.
The data from this study were initially presented to the ICH E14 Discussion Group at the 16 September 2014 teleconference and it demonstrated that exposure-response analysis in a small study of healthy volunteers could effectively detect mild QT prolongation. This study’s results, coupled with a decade of experience in exposure-response analysis for QT effects, suggest that QT assessment in early-phase clinical studies could serve as an alternative to the Thorough QT (TQT) study. A proposed criterion for a TQT waiver suggests that the upper bound of the two-sided 90% confidence interval of predicted placebo-adjusted QTc should be below 10 ms at the drug’s highest clinically relevant plasma concentrations.
While concentration-response profile plays a crucial role in comprehensively assessing the risk of QT prolongation, it is imperative to underscore that non-clinical data holds substantial significance as a key component within the totality of evidence framework and forms an integral part of the comprehensive approach to elucidating the impact of a prospective drug on the risk of arrhythmia. FDA led a global collaborative initiative, known as the Comprehensive In vitro Proarrhythmia Assay (CiPA), stemming from a workshop held in July 2013 at the US FDA. This assay serves as a risk prediction tool, relying on in vitro assessments of a drug’s impact on various types of channels in the muscle cells of the heart.
The CiPA methodology employs automated, high-throughput techniques, providing a thorough evaluation of a drug’s proarrhythmic potential by considering multiple cardiac ionic currents. This approach extends beyond assessing repolarizing currents alone, such as IKr, and utilizes in silico modeling to reconstruct the ventricular action potential. The goal is to gauge the propensity for Early Afterdepolarizations (EADs) and repolarization instability. This proposed mechanism-based strategy integrates data from multiple sources, including in vitro measurements and in silico modeling, with a confirmatory role for human stem cell–derived cardiomyocytes, which offer an integrated response from a human-based physiological system. The CiPA paradigm is not designed to reproduce arrhythmia but rather to directly assess risk liability based on mechanistic understanding of repolarization instability enabling the electrophysiological trigger for TdP proarrhythmia.
In conjunction with early-phase electrocardiogram (ECG) testing using the exposure-response approach, which includes a TQT study, and validation through more specific ECG-based biomarkers, the CiPA tool presents a novel regulatory approach. This approach has the potential to significantly decrease the attrition of potentially beneficial drugs in the development pipeline.
In 2015, ten years after the release of the ICH E14 (2005) and the ICH S7B (2005) guidelines, a new method called concentration-QTc (C-QTc) analysis was introduced via Questions and Answers (Q&As) in ICH E14. This method allows for the submission of C-QTc modeling results, in conjunction with nonclinical data, during a small Phase 1 study, as a substitute for a dedicated TQT study before progressing to Phase 1/2a trials.
A notable example showcasing the effective application of this Phase 1 C-QTc analysis approach is the study conducted for GLPG1972, also referred to as S201086, an ADAMTS-5 inhibitor. The study’s design was to mimic a Phase 1 trial for drugs that had already undergone a TQT study. They employed exposure-response modeling to assess QT prolongation. The results of this study were in line with the drug-induced changes in QT intervals observed in previous TQT studies. This demonstrated that electrocardiogram (ECG) assessments in Phase 1 studies could effectively replace the need for a dedicated TQT study.
This alternative approach, using C-QTc modeling, not only reduces the financial burden and time constraints associated with TQT studies, but also offers the significant benefit of reducing potential risks to study participants who might otherwise be exposed to exceptionally high doses of the investigational drug and moxifloxacin, which is commonly administered in typical TQT studies. However, it’s important to note that some medications, even if considered safe, may still undergo additional evaluation due to potential risks.
3. From 2020 to 2022:
In the process of developing new drugs, one of the major challenges is understanding how the results obtained from nonclinical tests predict the effects on the QT interval in humans. It’s essential to conduct integrated risk assessments that combine information from nonclinical and clinical data.
Over time, the ICH E14 guideline has seen significant updates, while the S7B guideline has remained relatively unchanged. The original ICH S7B guideline from 2005 outlined a nonclinical testing strategy to assess a drug’s potential to delay ventricular repolarization. This strategy involved two core studies: an in vitro hERG assay, which evaluates the drug’s effect on a specific ion channel (IKr), and an in vivo telemetry study typically conducted in nonrodent species like dogs or monkeys. However, the 2005 guideline did not provide detailed instructions on how to conduct these studies or criteria for determining their outcomes.
In 2020, a new draft Questions and Answers (Q&As) for the ICH E14/S7B guidelines were released. These draft Q&As aimed to propose a more integrated approach to assessing the risk of QT prolongation, with a stronger emphasis on nonclinical data. This approach sought to facilitate a more comprehensive evaluation of a drug’s potential impact on QT intervals, making use of both nonclinical and clinical data. The 2020 draft Q&As provided additional details about the requirements and interpretation of results for nonclinical studies, making it easier to compare findings across different drugs. These updates also stressed the importance of integrating both nonclinical and clinical data into a unified assessment.
Specifically, the 2020 draft Q&As delved deeper into the requirements for the in vitro hERG assay, highlighting the significance of standardized protocols and the inclusion of positive controls in the same experiments as the test drug. They also introduced criteria for determining a negative outcome, based on the margin between the drug’s half-maximal inhibitory concentration (IC50) and its exposure at the high clinical dose during steady-state conditions. Furthermore, the recommendations for conducting and reporting in vivo telemetry studies were refined in the 2020 draft Q&As. These revisions included guidelines for assessing both exposure and effects within the same animal and, where possible, the application of exposure-response modeling.
4. From 2022 till present
In August 2022, the finalized guidance for the industry, “E14 and S7B Clinical and Nonclinical Evaluation of QT/QTc Interval Prolongation and Proarrhythmic Potential—Questions and Answers,” was released. This guidance built upon the 2020 draft by offering a comprehensive framework for utilizing nonclinical assays as part of an integrated risk assessment. The ultimate goal was to reduce the need for extensive and costly “TQT” clinical studies, enhancing decision-making during the drug approval process.
The guidance highlighted integrated nonclinical and clinical QT/QTc risk assessment, which includes nonclinical data, the time course of QT prolongation, the magnitude of QT prolongation, categorical analyses of outliers, and certain adverse events in patients that can signal potential proarrhythmic effects.
Both the intersection-union test and the concentration-response analysis can estimate the maximum effect of a drug treatment on the QTc interval, but they are not used to test the same hypothesis. An integrated nonclinical and clinical QT/QTc risk assessment is particularly valuable when conducting a TQT study or concentration-QTc analysis isn’t feasible. This could arise under scenarios where a placebo-controlled comparison is not possible; safety considerations preclude administering supratherapeutic doses to obtain high clinical exposures and/or safety or tolerability prohibit the use of the product in healthy participants. Even without placebo and healthy participant dosing, this approach remains valuable.
In situations where it is not possible to evaluate the QT/QTc effects at high clinical exposure, an integrated risk assessment is particularly important that the nonclinical in vivo studies are conducted at exposures covering the high clinical exposure. An integrated QT/QTc risk assessment can also be particularly valuable for drugs with heart rate effects that could affect accurate QTc determination, advanced techniques like pacing or heart rate correction might be considered in nonclinical in vivo studies or in assessments within the intended patient population.
In general, when combining two or more drugs, a TQT study or intensive late-stage monitoring may not be necessary if individual component drugs have been shown not to affect QT/QTc intervals. For large proteins and monoclonal antibodies, which typically have a low likelihood of direct ion channel interactions, a TQT study is also not necessary, unless specific concerns arise from mechanistic considerations or data from clinical or nonclinical studies.
Why Choose BioPharma Services
These above-mentioned regulatory advancements on proarrhythmic risk assessment reflect the ongoing progress in guidelines aiming to streamline drug development by giving greater importance to nonclinical data and early phase clinical data when assessing heart-related risks. The determination of whether a TQT study or intensive late-stage monitoring is necessary should be made collectively based on mechanistic considerations or data from clinical and nonclinical studies. An alternative approach involves assessing the concentration-QT response during Phase 1 Single Ascending Dose (SAD) and/or Multiple Ascending Dose (MAD) programs, in conjunction with in vitro studies will not only alleviate the financial burden and time constraints associated with TQT studies but also provide the significant benefit of reducing potential risks to study participants who might otherwise be exposed to exceptionally high doses of the investigational drug. The ultimate objective is to maintain the quality and safety of the drug approval process while making it more efficient.
Written by: Vivian Zha, Clinical Pharmacology Scientist and John Oldenhof, Executive Vice President, Scientific Affairs.