Advancing RNA-Targeted Therapies: A Phase 1 Clinical Perspective on Oligos and Beyond
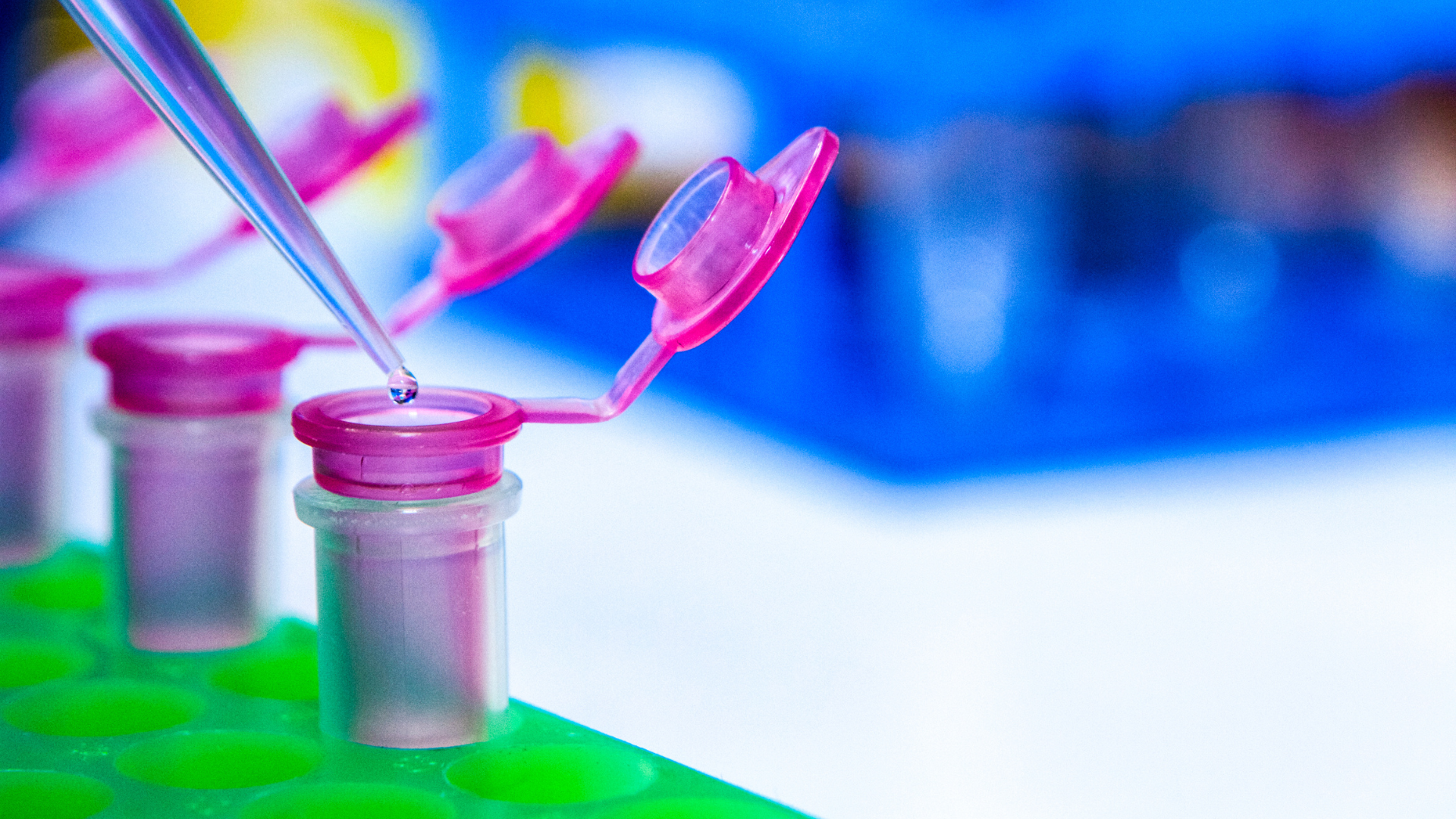
In recent years, RNA-targeted therapies have emerged as a promising frontier in the world of medicine. By harnessing the power of RNA, these innovative treatments have the potential to revolutionize how we treat a wide array of genetic diseases. As a leading Phase 1 clinical pharmacology unit, BioPharma Services plays a crucial role in advancing these therapies from the lab to the clinic, ensuring their safety and efficacy for the patients who need them most.
In this blog post, we will explore the exciting world of RNA-targeted therapies, including both oligonucleotide and non-oligonucleotide techniques, and delve into the crucial role that Phase 1 clinical trials play in their development.
RNA-Targeted Therapeutic Techniques
Over the past few decades, researchers have developed a diverse array of RNA-targeted therapeutic techniques to address various genetic diseases. These techniques work by modulating RNA molecules and their functions or use features of RNA in, offering unique approaches to treating conditions that were once considered untreatable.
Some RNA-targeted therapies have already been approved and include Antisense Oligonucleotides (ASOs), small interfering RNA (siRNA), Splice-Switching Oligonucleotides (SSO), aptamer, and messenger RNA (mRNA) (see Table below). Strategies in development include micro RNA (miRNA) and STOKE’s Targeted Augmentation of Nuclear Gene Output (TANGO) strategy. Other emerging RNA-targeted therapy techniques, such as CRISPR-Cas9-mediated gene editing (using a “programmable” RNA guide molecule) also hold great potential.
Advancements in Oligonucleotide RNA-Targeted Therapies
Oligonucleotide therapies, such ASOs, SSOs siRNA and aptamers have made remarkable strides in recent years, showing substantial promise in treating a range of genetic diseases and conditions.
Antisense Oligonucleotides (ASOs) are short, synthetic pieces of DNA or RNA that bind to specific messenger RNA (mRNA) sequences. This binding can block the translation of the mRNA into protein and/or lead to the degradation by RNase effectively down-regulating the mRNA expression into protein. Inotersen (Tegsedi®) approved for the treatment of hereditary transthyretin-mediated amyloidosis in 2018 is a 20-mer that binds to mRNA, leading to RNAse H1 degradation.
Splice Switching Oligonucleotides (SSO) also bind to mRNA and in this case disrupt the normal splicing process. This strategy can decrease expression if splicing is necessary for a functional protein. On the other hand, this strategy can also be used to increase expression of a functional protein in case altered splicing limits expression.
For example, Nusinersen (Spinraza®), an SSO, is used to treat Spinal Muscular Atrophy (SMA), a severe genetic disorder that affects muscle strength and movement. Nusinersen changes the splicing of SMN2, a backup gene for SMN1, which is mutated in SMA, leading to increased production of full-length SMN protein. This change is disease-modifying, improving motor function in SMA patients.
Another example is Eteplirsen (Exondys 51®) is a Splice-Switching Oligonucleotide used to treat Duchenne Muscular Dystrophy (DMD). It causes exon skipping, a form of mRNA splicing, which allows producing a partially functional dystrophin protein, thereby slowing disease progression.
RNA aptamers are short, single-stranded RNA molecules that can bind to specific target molecules. Although they do not target RNA, they are RNA oligos. They function much like antibodies, but are much smaller and easier to produce. They have potential applications in both diagnostics and therapeutics. Pegabtanib (Macugen®) approved in 2004 uses this strategy to bind to the 165 isoform of VEGF and works as antagonist to treat age-related macular degeneration (AMD).
Despite the success of these RNA-Targeted therapies, several challenges remain. Off-target effects can occur when the oligonucleotide binds to an unintended mRNA sequence, leading to the down regulation of a wrong gene. These effects can result in unintended side effects, which can range from mild to severe, depending on the gene affected.
Potential toxicities also present a challenge. For example, with repeated high-dose administration, some ASOs have been associated with kidney toxicity and thrombocytopenia (a decrease in platelets). In Phase 1 trials, these potential toxicities should be closely monitored through regular blood tests and other appropriate assessments.
Delivery hurdles are another challenge, as ensuring the oligonucleotides reach the correct cells and tissues in the body can be difficult. This is particularly a challenge for diseases affecting the brain, like SMA or DMD, due to the blood-brain barrier.
To overcome these challenges, researchers are refining the design and delivery methods of oligonucleotides. This includes chemical modifications to improve stability and specificity, and the use of nanoparticles or other delivery vectors to help the oligonucleotides reach their intended targets. Additionally, further understanding of the biology of these diseases and the pharmacokinetics and pharmacodynamics of these therapies can help in designing better molecules and delivery systems.
Phase 1 clinical trials are instrumental in addressing these challenges, helping to identify potential safety concerns, determine optimal dosing regimens, and monitor for any adverse effects or toxicities. Through rigorous Phase 1 trials, we can ensure that the promise of oligonucleotide therapies is realized safely and effectively.
Promising Non-Oligo RNA-Targeted Therapies
While oligonucleotide therapies have garnered much attention, other RNA-targeted therapies also show great promise and are transforming the landscape of genetic disease treatment.
Small Interfering RNAs (siRNA) are naturally occurring non-coding short double-stranded RNA nucleotides. They operate in the RNA interference pathway. RNA interference (RNAi) is a natural biological process that regulates gene expression. Scientists have harnessed this mechanism to develop therapies that can silence disease-causing genes. Patisiran (Onpattro®amy) is an example of a successful RNAi therapy. It’s used for the treatment of hereditary transthyretin-mediated amyloidosis, a rare and often fatal genetic disease. Patisiran works by degrading the mRNA for transthyretin, preventing the production of the faulty protein and slowing the progression of the disease.
Small Activating RNAs (saRNAs) work by a different mechanism, upregulating gene expression rather than downregulating it. They target promoter regions of genes, stimulating transcription and leading to increased production of the desired protein.
MicroRNAs (miRNAs) are naturally occurring small non-coding RNAs that can regulate gene expression post-transcriptionally. They can be mimicked or inhibited to modulate the expression of their target genes, providing another avenue for disease treatment. Miravirsen, an miRNA inhibitor, is in development for the treatment of hepatitis C. It works by sequestering miR-122, a liver-specific miRNA essential for hepatitis C virus replication.
STOKE’s TANGO strategy takes a unique approach to RNA modulation to increase protein expression in case of haploinsuffiency. It uses antisense oligonucleotides to increase the expression of the functional copy of the targeted protein. Through this strategy, they overcome the deficiency in expression of the mutated copy of the gene that may produce deficient mRNA that is degraded or produces a non-functional copy of the protein. The oligo binds to a site on the pre-mRNA that removes an NMD (“poison”) exon and so promotes the generation of more functional mRNA. This strategy is currently in development for the treatment of Dravet Syndrome, a severe form of epilepsy.
STOKE Therapeutics is using this approach to develop TANGO (Targeted Augmentation of Nuclear Output) therapies for genetic diseases, like Dravet Syndrome, caused by insufficient protein production.
CRISPR-Cas9 gene editing has profoundly transformed the field of genetic engineering, enabling precise alterations to DNA sequences within a genome. This technique can add, remove, or modify sections of these sequences, presenting immense potential for treating a diverse array of genetic diseases. Central to its operation is its use of guide RNAs (gRNAs), molecules of RNA that are designed to bind to specific DNA sequences.
These gRNAs guide the Cas9 enzyme to the target sequence in the DNA, acting as a GPS system of sorts. The Cas9 enzyme then makes a cut at the target location, enabling the addition, deletion, or alteration of DNA. This unique utilization of RNA to target DNA sequences underscores CRISPR-Cas9’s relevance in the panorama of RNA techniques, highlighting the interplay between these two fundamental biomolecules in advancing genetic engineering.
Ribozymes are RNA molecules that have catalytic activity and can perform chemical reactions. These molecules can be engineered to target and cleave specific RNA sequences, providing a method for downregulating gene expression.
RNA vaccines, such as Pfizer-BioNTech’s BNT162b2 and Moderna’s mRNA-1273, have demonstrated the transformative potential of RNA-based therapeutics. These vaccines use mRNA to instruct cells to produce a piece of the spike protein found on the surface of the SARS-CoV-2 virus. The immune system recognizes this protein as foreign and mounts an immune response against it. This approach was key in the rapid development of effective vaccines during the COVID-19 pandemic and has significant implications for the future of vaccine development.
These non-oligonucleotide RNA-targeted therapies are expanding the frontier of genetic disease treatment. They bring with them the promise of new treatments that are more targeted, effective, and adaptable to a wide range of diseases. However, like all therapies, they come with their own set of challenges such as off-target effects, immune responses, and delivery issues. Phase 1 clinical trials play a pivotal role in overcoming these hurdles, ensuring the safety and efficacy of these promising therapies.
The Future of RNA-Targeted Therapies and Phase 1 Trials
As RNA-targeted therapies continue to advance, they are set to reshape the landscape of modern medicine, offering hope for patients with genetic diseases who have limited or no treatment options. However, a key stage in the development of these therapies is the successful completion of Phase 1 clinical trials.
Phase 1 trials represent a critical juncture in the drug development process. These early-stage studies help to identify the safety profile of a new therapy, determine the appropriate dosage, and uncover potential side effects. These trials are especially important when it comes to RNA-targeted therapies, given their unique mechanisms of action and potential challenges.
Given the novel nature of these therapies, Phase 1 trials must be meticulously designed to thoroughly assess safety and tolerability. This includes careful monitoring for potential off-target effects and immune responses, two of the more common challenges associated with RNA-targeted therapies. The nature of the therapy may also require specialized delivery methods, such as intrathecal or intravenous administration, which must be factored into the trial design.
Another unique consideration for Phase 1 trials of RNA-targeted therapies is their potential for rapid efficacy. Unlike many other therapies that can take weeks or months to show an effect, RNA-targeted therapies can often show an effect within days. This means that Phase 1 trials may offer early signs of efficacy, providing valuable information to guide the design of later-stage trials.
In addition, Phase 1 trials of RNA-targeted therapies need to consider the specific disease being targeted. Some genetic diseases are rare, meaning that patient recruitment can be challenging. Additionally, the severity and progression of the disease can vary widely among patients, which may affect the trial outcomes.
As such, the design and execution of Phase 1 trials for RNA-targeted therapies require careful planning and expertise. Despite the challenges, the potential of these innovative treatments to change the course of genetic diseases makes the journey worthwhile. Through rigorous Phase 1 trials, we can ensure the safety and efficacy of these therapies, bringing us one step closer to a future where genetic diseases can be effectively treated or even cured.
Healthy Volunteers vs. Patients: Strategic Considerations for Phase 1 Trials
When designing Phase 1 trials, a crucial question to consider is whether to conduct the study in healthy volunteers or in patients with the disease the therapy is intended to treat. The answer to this question depends on several factors, including the nature of the therapy, the disease being targeted, and the potential risks and benefits to the participants.
For many traditional drug therapies, Phase 1 trials are typically conducted in healthy volunteers. This allows researchers to study the therapy’s pharmacokinetics (how the drug is processed by the body) and pharmacodynamics (the effects the drug has on the body) without the confounding effects of an underlying disease. It also allows for the identification of potential side effects in a controlled setting where participants are not already dealing with illness symptoms.
However, for RNA-targeted therapies, the decision is often more complex. RNA-targeted therapies tend to be highly specific to certain genetic sequences, meaning they may not have any effect in healthy individuals who do not carry the relevant genetic mutation. In these cases, it may be more appropriate to conduct Phase 1 trials in patients with the disease.
Additionally, some RNA-targeted therapies are intended to treat severe or life-threatening diseases for which no other treatments are available. For these therapies, the potential benefits may outweigh the risks, and it may be considered ethical to conduct Phase 1 trials in patients, despite the experimental nature of the therapy.
On the other hand, RNA-targeted therapies can also carry risks, including off-target effects and immune responses. If these risks are deemed significant, it may be more appropriate to conduct initial safety studies in healthy volunteers. In such cases, careful monitoring and a thorough informed consent process are essential to ensure the volunteers understand the experimental nature and potential risks of the therapy.
Furthermore, practical considerations may also come into play. For rare genetic diseases, finding a sufficient number of patients for a Phase 1 trial can be challenging. In such cases, researchers might opt to start with a trial in healthy volunteers to gather initial safety and dosing data before moving to a patient population.
In summary, the decision to conduct Phase 1 trials in healthy volunteers or patients depends on a careful balancing of the scientific, ethical, and practical considerations associated with each particular RNA-targeted therapy. As these therapies continue to evolve, so too will the strategies for their testing, with the ultimate goal of ensuring safety and efficacy for the patients who stand to benefit from them.
Looking Into the Future of RNA-targeted Therapies
As we look to the future, the role of RNA-targeted therapies in medicine is undoubtedly set to grow. From antisense oligonucleotides to RNA interference, miRNA modulation of expression, and beyond, these innovative treatments offer new hope for patients with genetic diseases. However, it’s crucial to remember that the journey of these therapies from the lab to the clinic is not a straightforward one.
Phase 1 clinical trials play a vital role in this journey, helping to ensure that these promising therapies are safe, effective, and ready for the patients who need them most. At BioPharma Services, we’re proud to be part of this exciting field, working tirelessly to advance the next generation of RNA-targeted therapies and bring new treatments to the patients who need them most.
We hope you enjoyed this journey through the exciting landscape of RNA-targeted therapies. Stay tuned for more updates from the cutting-edge world of Phase 1 clinical trials and the revolutionary therapies we’re helping to advance. We’re just getting started, and we can’t wait to see what the future holds.
Written By: Dr. John Oldenhof, Executive Vice President, Scientific Affairs.
Table: Summary of RNA based therapies
Technique | Mechanistic Summary | Pros | Cons | Common Side Effects | Suitable for Phase 1 in Healthy Volunteers | Current Approved Examples (Agency) |
Antisense Oligonucleotides (ASOs) | Bind to mRNA and prevent translation often by RNase degradation | Targeted, versatile | Potential off-target effects, delivery challenges | Flu-like symptoms, injection site reactions | Yes, depending on disease and therapy specifics |
Fomivirsen (Vitravene®) 1998, Mipomersen (Kynamro®, FDA) 2013, Inotersen (Tegsedi®, FDA) 2018, Volanesorsen (Waylivra®, FDA) 2019 |
Short inhibitory RNAs (siRNAs) | Bind to mRNA and induce degradation | Targeted, potent | Delivery challenges, potential off-target effects | Injection site reactions, hypersensitivity reactions | Typically no due to specificity |
Patisiran (Onpattro, FDA) 2018, Givosiran (Givlaari, FDA) 2019, Lumasiran (Oxlumo, FDA) 2020, Inclisiran (Leqvio® EMA, FDA) 2020 and 2021, Vuttrisiran (Amvuttra®, FDA) 2022 |
mRNA | Mimic endogenous mRNA and produces entire or part of protein | Targeted | Delivery challenges pending target cells | Varies depending on target and delivery method | Yes | Not yet approved |
miRNA Mimics and Antagomirs | Mimic or inhibit endogenous miRNAs | Targeted, versatile | Delivery challenges, potential off-target effects | Varies depending on target and delivery method | Typically no due to specificity | Miravirsen (Clinical trials, not yet approved) |
Splice-Switching Oligonucleotide (SSO) | Bind to pre-mRNA and modulate splicing | Targeted, versatile | Potential off-target effects, delivery challenges | Injection site reactions, flu-like symptoms | Yes, depending on disease and therapy specifics |
Nusinersen (Spinraza®, FDA) 2016, Eteplirsen (Exondys® 51, FDA) 2016, Golodirsen (Vyondys® 53, FDA) 2019 Viltolarsen (Viltepso®, FDA) 2020, Casimersen (Amondys 45®, FDA) 2021 |
RNA Aptamers | Bind to target proteins and modulate their activity | Targeted, flexible | Potential off-target effects, immune responses | Varies depending on target and delivery method | Yes, depending on disease and therapy specifics | Pegaptanib (Macugen®, FDA) 2004 |
RNA Vaccines | Encodes antigenic proteins that stimulate an immune response | Rapid development, potent | Potential immune responses, cold chain storage for some formulations | Pain at injection site, fever, fatigue | Yes, as seen in COVID-19 vaccine trials |
Tozinameran (Comirnaty® FDA) 2021, Elasomeran (Spikevax®, FDA) 2022 |
CRISPR-Cas9-mediated gene editing | Induces double-strand breaks in DNA, guided by RNA sequence leading to gene editing | Potent, versatile | Potential off-target effects, immune responses | Varies depending on target and delivery method | Typically no due to specificity | Not yet approved |
TANGO (Targeted Augmentation of Nuclear Gene Output) | Oligo binding to exon in pre-mRNA to produce more functional mRNA that leads in increase in functional protein expressoin | Targeted, versatile | Technically challenging, potential off-target effects, dependent on presence of exon | Not yet known | Investigated for severe epilepsies | In clinical trials for Dravet. |
Please note that the suitability for Phase 1 trials in healthy volunteers is a general guideline and can vary depending on the specifics of the therapy and the disease. Also, the approved examples column lists some of the major approvals
BioPharma Services, Inc., a Think Research Corporation and clinical trial services company, is a full-service Contract Clinical Research Organization (CRO) based in Toronto, Canada, specializing in Phase 1 clinical trials 1/2a and Bioequivalence clinical trials for international pharmaceutical companies worldwide. BioPharma has clinical facilities both in the USA and Canada with access to healthy volunteers and special populations.